Neutron stars are the incredibly dense remnants of massive stars that have undergone a fiery collapse known as a supernova. The tiniest and densest known class of stellar objects are neutron stars, except for black holes. It is believed that the primary component of these incredibly dense and compact stars is neutrons.
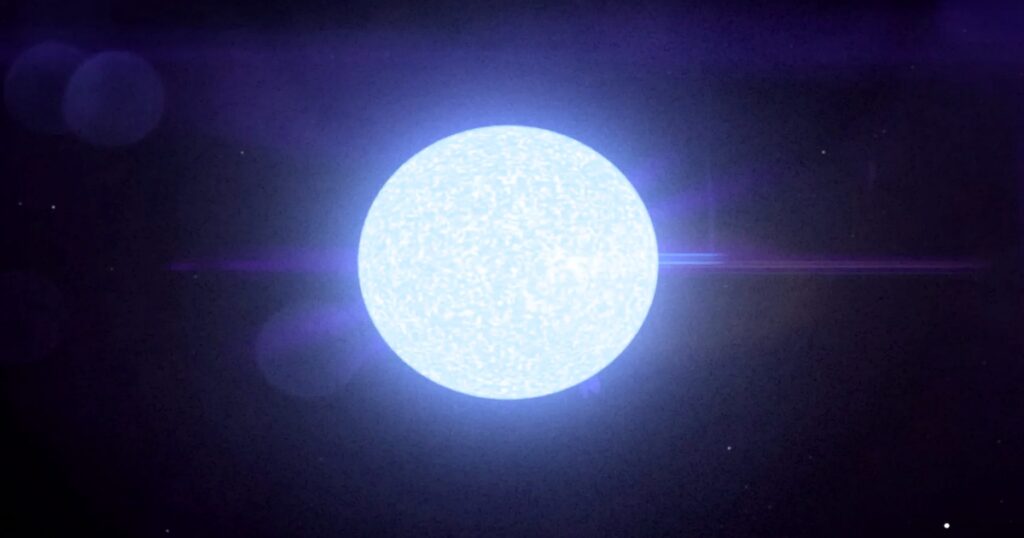
Most of them have masses of 1.35 times that of the Sun, although they range from 1.18 to 1.97 times that of the Sun. As a result, they have incredibly high mean densities, roughly 1014 times that of water. This is similar to the density seen inside the nucleus of an atom, and one may conceptualize a neutron star as a massive nucleus.
They arise from the combination of gravitational collapse and a large star’s supernova explosion, which compresses the core beyond the density of a white dwarf star to the density of atomic nuclei.
Since neutron stars are among the last phases of the life cycle of huge stars, a star corpse can theoretically live “forever.” Nevertheless, they may eventually combine and form a black hole or a more massive neutron star if they have a binary companion, such as another neutron star or black hole.
What is the Size of Neutron Stars?
Despite their immense mass, neutron stars are surprisingly compact. Their radius typically falls within a range of 10 to 15 kilometers (6 to 9 miles). Imagine a sphere roughly the size of a large city, yet containing the mass of our Sun.
Given the radius, we can calculate the diameter of a neutron star by multiplying the radius by 2. So, a typical neutron star would have a diameter ranging from 20 to 30 kilometers (12 to 18 miles).
What is the Mass of Neutron Stars?
Neutron Stars typically pack a mass that is 1.4 to 2.1 times the mass of our Sun. This incredible mass is crammed into their tiny radius, resulting in incredibly high densities.
What is the Volume of Neutron Stars?
Due to their compact size, the volume of a neutron star is minuscule compared to our Sun. Imagine shrinking a giant beach ball down to the size of a marble. That’s roughly the difference in volume between a neutron star and the Sun.
What is the Density of Neutron Stars?
They pack the mass of our Sun into a sphere that would barely cover a major city. This density reaches up to 100 trillion times that of water. A teaspoon of neutron star material would weigh a staggering 100 billion tons, exceeding the mass of Mount Everest.
The reason behind this extreme density lies in the star’s composition. Unlike regular stars made up of atoms with protons, electrons, and neutrons, neutron stars are primarily composed of neutrons.
The immense pressure during the supernova fuses protons and electrons together, creating a sea of neutrons packed tightly within the star. Neutrons, lacking electrical charge, can snuggle up against each other without repulsion, allowing for this incredibly compact structure.
What is the Temperature of Neutron Stars?
When a neutron star is first born from a supernova, it possesses a scorching temperature, reaching billions of degrees Celsius. However, over time, neutron stars cool down through various processes like neutrino emission and thermal radiation. The exact temperature of a neutron star depends on its age and can vary significantly.
What is the Composition of Neutron Stars?
Predominantly Neutrons: As the name suggests, neutron stars are primarily composed of neutrons, crammed together at unimaginable densities. During the supernova that birthed the neutron star, the immense pressure overcame the natural repulsion between protons and electrons in atoms, forcing them to combine and form neutrons.
However, neutron stars aren’t entirely pure neutronium (a hypothetical state of pure neutrons). They likely harbor a small percentage of protons and electrons, particularly in the outer crust. Additionally, depending on the specific conditions during the supernova, some exotic particles like hyperons (containing strange quarks) might be present.
What is the Interior of Neutron Stars?
Outer Crust:
The outermost layer, estimated to be a few kilometers to tens of kilometers thick, is thought to be a solid lattice of nuclei (mostly iron nuclei) with electrons squeezed in between. This region experiences the least pressure compared to the deeper layers.
Inner Crust:
As we move inwards, the pressure intensifies. The nuclei become more exotic, potentially containing neutrons and protons in addition to iron. This layer might exhibit a fascinating “nuclear pasta” structure, with various shapes formed by the dense nuclear matter.
Outer Core:
Deeper still lies the outer core, a region where the density becomes truly mind-boggling. Here, the distinction between individual nuclei and electrons blurs. The matter might exist in a superfluid state, where neutrons flow freely with minimal friction.
Inner Core (Uncertain Realm):
The exact nature of the inner core remains a mystery. Some theories suggest it could be composed of superfluid neutron matter, similar to the outer core, but at even higher densities. Other possibilities include a state of quark-gluon plasma, where the fundamental particles, quarks and gluons are no longer confined within protons and neutrons.
What does the Atmosphere of Neutron Stars consist of?
Unlike stars that generate light and heat through nuclear fusion, neutron stars have a very thin and tenuous atmosphere. This atmosphere is likely composed of hydrogen, helium, and heavier elements ripped away from the companion star in binary systems or left over from the supernova explosion.
The composition and density of the atmosphere can vary significantly depending on the specific neutron star and its environment. Some might have a nearly undetectable atmosphere, while others might have a more substantial envelope.
Neutron Stars’ Formation:
Neutron Stars originate from the dramatic deaths of colossal stars, at least eight times more massive than our Sun. As these giants reach the end of their fuel reserves, gravity takes over, crushing the core inwards and triggering a spectacular supernova explosion.
The supernova blasts the star’s outer layers outward, enriching the universe with heavier elements forged within the star’s core.
The leftover core experiences such immense gravitational pressure during the supernova that it overcomes the natural repulsion between protons and electrons in atoms. This pressure forces them to combine, forming a neutron-rich core, the very essence of a neutron star.
How Strong is Neutron Stars’ Magnetic Field?
A neutron star has a magnetic field millions to billions of times stronger than Earth’s. The immense gravitational crush during the supernova not only squeezes the core into a neutron-rich state, but it also twists and amplifies the star’s existing magnetic field.
These fields become incredibly strong, rivaling the most powerful magnetic structures ever observed.
The consequences of these super-charged magnetic fields are dramatic. They can:
Channel Charged Particles:
The powerful magnetic fields act like cosmic funnels, channeling streams of charged particles like electrons and positrons around the star’s poles. These energetic particles can interact with surrounding material, producing powerful beams of radiation.
Shape the Environment:
The intense magnetic fields extend far beyond the neutron star itself, influencing the environment around it. In some cases, they can even interact with the material ripped away during the supernova, creating spectacular features like nebulae.
How Fast is Neutron Stars’ Spinning Speed?
Another fascinating property of neutron stars is their incredibly rapid rotation. During the supernova explosion, the collapsing core loses a significant portion of its mass. However, the star’s angular momentum, a measure of its spinning motion, is conserved.
As the core shrinks dramatically, this angular momentum gets concentrated, causing the neutron star to spin incredibly fast. Some neutron stars can rotate hundreds of times per second, To put that in perspective, our Sun rotates on its axis roughly once every 27 days.
The fastest-spinning neutron star ever discovered, aptly named PSR J1748-2446ad, boasts a phenomenal rotation speed of 716 times per second! This translates to a rotational period of just 1.4 milliseconds, meaning it completes one full spin in a mere blink of an eye.
Types of Neutron Stars:
Based on some unique properties, Neutron Stars are divided into two types, Pulsars & Megnatars. We will discuss the difference between Pulsars and Magnetars.
Pulsars versus Magnetars
What are Pulsars?
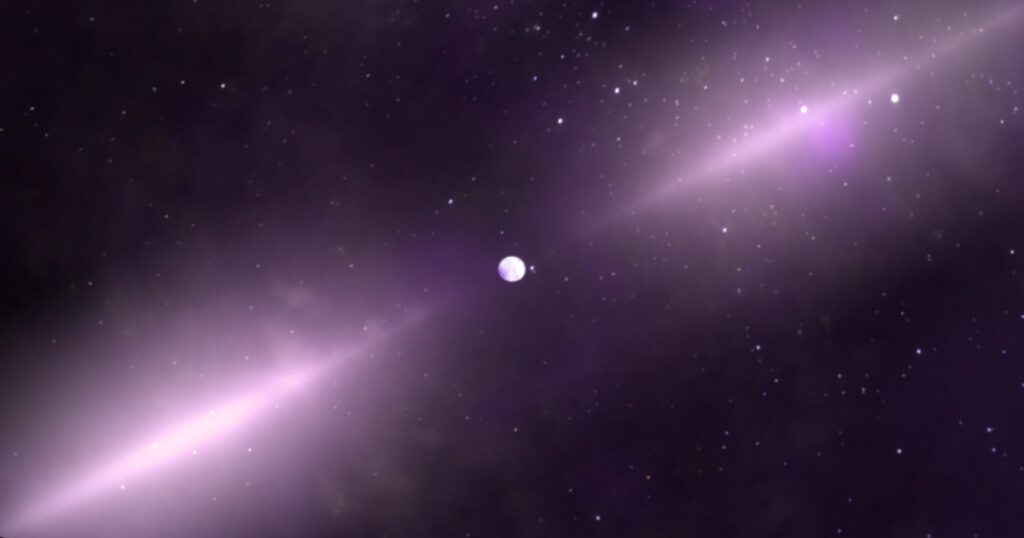
Pulsars are highly magnetized, rotating neutron stars that emit beams of electromagnetic radiation along their magnetic poles. These beams are observable as pulses of radiation that are detected at regular intervals, hence the name “pulsars.”
Magnetic Field Strength:
While pulsars boast powerful magnetic fields, millions to billions of times stronger than Earth‘s, they are considered the “weak sisters” compared to magnetars.
Emission Signature:
The defining characteristic of a pulsar is its regular, pulsed emission of radiation, typically radio waves. This pulsating behavior arises from the interplay between the star’s strong magnetic field and rapid rotation. As the magnetic field sweeps past our line of sight, we observe a pulse of radiation.
Activity and Stability:
Pulsars are generally considered to be more stable objects. Their rotational period, the time it takes for one complete spin and emission cycle, remains relatively constant over time.
What are Magnetars?
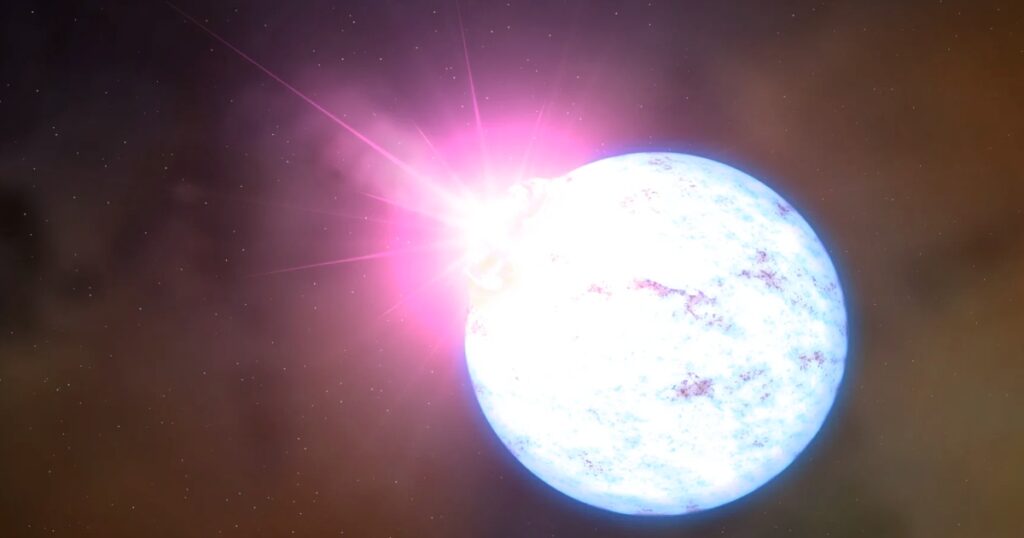
Magnetars are a type of neutron star with extremely powerful magnetic fields, thousands of times stronger than typical neutron stars. They are characterized by sporadic bursts of X-rays and gamma rays, believed to result from intense magnetic activity within their structure.
Magnetic Field Strength:
These celestial titans hold the crown for the strongest magnetic fields ever observed in the universe. Estimates suggest their magnetic fields are millions of times stronger than those of a typical pulsar.
Emission Signature:
Magnetars can exhibit a wider range of emission behaviors. While some may show pulsar-like pulsations, their ultra-strong magnetic fields can also lead to sudden, dramatic releases of energy in the form of powerful X-ray and gamma-ray flares. These flares can be much more energetic than the emissions observed from pulsars.
Activity and Stability:
Due to their ultra-strong magnetic fields, magnetars can be much more active and unpredictable. The immense magnetic stress can cause their crusts to crack and rearrange, releasing bursts of energy called magnetar starquakes.
These quakes, in turn, can release even more energy and energetically erupt radiation from their surface, resulting in superflares that dwarf even the most powerful flares observed from regular stars. These “starquakes” and superflares can disrupt the otherwise regular rotation of a magnetar, making their emission patterns less stable compared to pulsars.
Feature | Pulsar | Magnetar |
---|---|---|
Formation | Most common outcome of a supernova | Rare, theorized from especially massive stars |
Magnetic Field Strength | Millions to billions of times Earth’s | Trillions of times Earth’s, strongest known |
Emission Patterns | Pulsating beams of radio waves, X-rays, or gamma rays | Pulsations, but also monstrous flares |
When and Who Discovered Neutron Stars?
Concept:
The concept of neutron stars emerged in the theoretical realm long before they were directly observed. In 1932, Sir James Chadwick discovered the neutron, a fundamental particle with no electrical charge. Just a year later, in 1933, Walter Baade and Fritz Zwicky, pondering the immense energy released during a supernova explosion, proposed the existence of neutron stars.
They theorized that the immense gravitational collapse during a supernova could squeeze protons and electrons together, forming neutrons and resulting in an incredibly dense star, the neutron star.
Breakthrough:
For decades, the existence of neutron stars remained purely theoretical. However, in 1967, a groundbreaking discovery changed the game. Jocelyn Bell Burnell, a graduate student at the time, detected strange, rapidly pulsating radio signals while studying distant celestial objects.
These pulsating signals, initially dubbed “Little Green Men” signals due to their unexplained nature, were eventually attributed to a new type of celestial object: the pulsar. Pulsars are neutron stars with strong magnetic fields and rapid rotation that emit beams of radiation, like radio waves, in a highly regular and pulsed manner.
The discovery of pulsars provided the first concrete evidence for the existence of neutron stars. Since then, astronomers have identified thousands of pulsars, each offering valuable insights into the properties and behaviors of neutron stars.
Different Types Emerge:
As astronomers delved deeper into the world of neutron stars, they discovered a surprising diversity. Pulsars themselves come in various flavors, with different spin rates and emission properties. Additionally, the study of pulsars led to the identification of other fascinating types of neutron stars:
Magnetars: These celestial powerhouses boast ultra-strong magnetic fields, millions of times stronger than those of a typical pulsar. Magnetars can exhibit dramatic outbursts in the form of powerful X-ray and gamma-ray flares.
Binary Systems: Many neutron stars are found in binary systems, where they orbit another star. Studying these systems allows astronomers to measure the mass of the neutron star and learn more about its interaction with its companion.
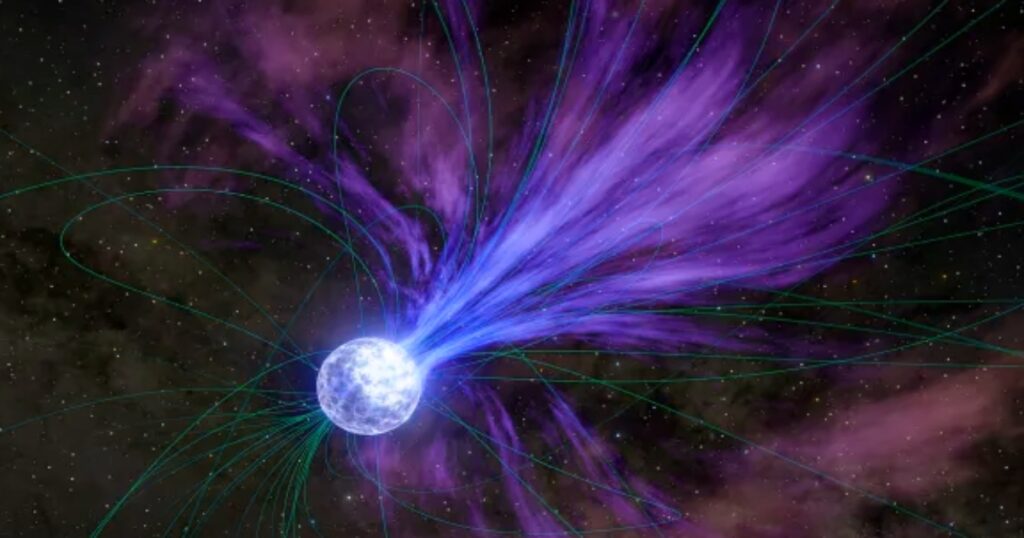
What are the Examples of Neutron Stars?
The Crab Nebula Pulsar:
This iconic pulsar is the remnant of a supernova observed in 1054 AD. It boasts a rotation rate of 30 times per second and is a powerful source of radio waves and other forms of radiation.
J0740+6620:
This double neutron star system holds the record for the fastest-spinning pulsars ever discovered, with each star rotating hundreds of times per second.
SGR 1806-20:
This magnetar is infamous for a giant flare it unleashed in 2004, the brightest flare ever detected from outside our Milky Way galaxy.
What is the Importance of Neutron Stars?
Neutron stars, beyond their awe-inspiring nature, play a crucial role in the grand story of the universe. They serve as cosmic laboratories for studying physics under extreme conditions, contribute to the creation of heavier elements, and might even be linked to some of the most energetic phenomena observed, gamma-ray bursts.
Imagine a place where gravity crushes matter to unimaginable densities, magnetic fields reach mind-boggling strengths, and particles spin at dizzying speeds. That’s the reality within a neutron star. These extreme conditions make them invaluable laboratories for studying physics beyond anything achievable on Earth.
Here’s how neutron stars shed light on fundamental forces:
Ultra-Dense Matter:
The incredible density of neutron stars allows us to explore how matter behaves under extreme pressure. This knowledge helps us understand the behavior of neutron stars themselves and might even hold clues about the nature of dark matter, a mysterious substance thought to dominate the universe’s mass.
Super-Strong Magnetic Fields:
The powerful magnetic fields within neutron stars are unlike anything found elsewhere. Studying how these fields interact with matter and radiation helps us understand fundamental forces like electromagnetism on a whole new level.
Rapid Rotation and General Relativity:
The dizzying rotation of neutron stars pushes the boundaries of Einstein’s theory of general relativity. By studying their behavior, we can test the limits of our current understanding of gravity and space-time itself.
Heavyweight Element Formation:
When two neutron stars collide, the immense energy released can forge heavier elements, like gold and platinum, that are not readily created in regular stars. These collisions are thought to be a major source of the heavier elements found throughout the universe, including those that make up our planet and even our own bodies.
Gamma-Ray Burst Connection:
Gamma-ray bursts are some of the most energetic explosions observed in the universe. While the exact mechanism is still under debate, some theories suggest that the collision of neutron stars or the collapse of a very massive star into a neutron star could be responsible for these powerful bursts.
What are the Challenges of Observing Neutron Stars?
Unfortunately, directly observing neutron stars is no easy feat. Unlike stars that shine brightly due to nuclear fusion, most neutron stars are faint and difficult to detect. However, astronomers have developed clever ways to study these elusive objects:
Pulsars as Beacons:
Pulsars, with their regular emissions, act like cosmic lighthouses. By studying their pulsations, astronomers can learn about the rotation rate, magnetic field strength, and even the presence of companion stars orbiting the neutron star.
Telescopes and Instruments:
Powerful telescopes and instruments operating across various wavelengths of the electromagnetic spectrum, including X-ray and gamma-ray observatories, are crucial for detecting faint emissions from neutron stars and their surrounding environments.
Binary Systems:
When a neutron star resides in a binary system with another star, the interaction between them can reveal valuable information about the neutron star’s properties, such as its mass.
Conclusion:
From fiery birth to crushing density, neutron stars are cosmic marvels. Packed with extremes, they act as laboratories for physics & birth elements vital for life. Unveiling their secrets deepens our understanding of the universe’s wonders.